Periodic Table
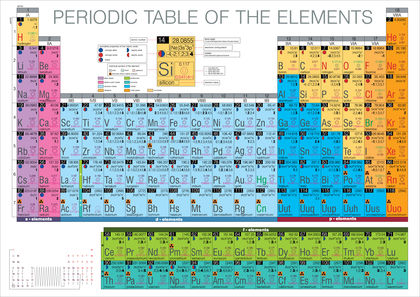
The Periodic Table places the symbols of chemical elements, sequenced by atomic number , in rows and columns that align similar properties.
Antiquity through the Renaissance
A few thousand years ago, primitive chemistry focused mostly on converting one substance into another. The word "chemistry" itself is arguably traced to the name of a region of ancient Egypt where such transformation attempts were practiced. Over the centuries, philosophers tried to come to terms with the growing variety of known substances. They postulated the role of fundamental entities that could not be broken down further but formed simple materials when combined. By the time of ancient Greece, Democritus, Leucippus, and Empedocles expounded the nature of matter in terms of constituent elements, the simple substances—earth, air, fire, and water—of which all materials were compounded. The term "atom" first appears in this context.
A millennium or so later, Arab civilizations made great strides in laboratory techniques. Subsequently, during the Renaissance period, these techniques were adopted in trying to transform one element into another, most notably into gold from less costly substances like lead. This gave the Arabic term "alchemy" its modern mystical connotation.
Post-Renaissance
By the mid-1700s about twenty elements were known. Science was beginning to get more sophisticated as measurements and instrumentation improved rapidly and theories based on observation grew more advanced and more compelling. Chemists, however, continued to anguish over the inability to easily categorize the elements.
What was likely the first attempt at sorting the elements was a table of simple substances, prepared in 1772 by French chemist Louis-Bernard Guyton de Morveau. French chemist Antoine-Laurent Lavoisier was most influential in developing an experimental approach, which is acknowledged to have laid the foundation for modern chemistry. In 1789, Lavoisier published a list of pure substances that included the known elements but also some compounds and light and heat. By the early 1800s, following the introduction of English chemist John Dalton's atomic theory and the concept of atomic weights, the number of known elements had grown. Although properties were carefully measured, confusion held sway when it came to agreeing on the composition of compound substances and the related assignment of atomic weights.
In 1829 German chemist Johann Döbereiner noted that there were triplets of elements in which the central species' properties were almost exactly midway between the outer two. The first example of such a triplet included chlorine, bromine, and iodine. Properties such as atomic weights, color, and reactivity followed this "law of triads" for several such groupings, but not for the entire collection of known elements.
In 1860 Italian chemist Stanislao Cannizzaro presented analyses at an international chemistry meeting that, when merged with previously ridiculed hypotheses by fellow Italian Amedeo Avogadro, yielded unambiguous atomic weights. These eliminated most of the disharmony among property determinations. In attendance were German chemist Lothar Meyer and Russian chemist Dimitri Mendeleev, both of whom were inspired to give the presentation further thought.
Industrial Age
In 1862 French geologist Alexandre-Émile Beguyer de Chancourtois arranged the elements in order of increasing atomic weights, wrapping the series around a cylinder in a helical display. He noted that elements with similar properties lined up, one over the other. His idea was obscured by its publication in a nonchemistry journal, the inclusion of compounds and alloys in the discussion, and the publisher's decision not to include an essential diagram.
JOHN NEWLANDS (1837–1898)
John Newlands compared elements to musical notes with his law of octaves. As on a scale, every eighth element would share similar properties when arranged by increasing atomic weight. Newlands did not account for exceptions, however, and it was only upon establishment of the Periodic Table that his theory gained credibility.
—Valerie Borek
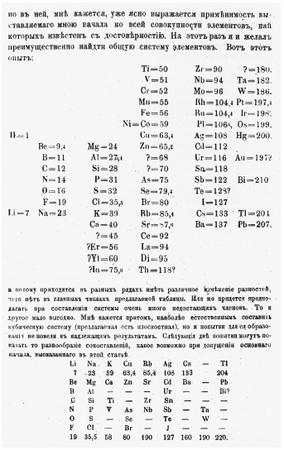
A few years later, British chemist John Newlands also arranged the elements in order of increasing atomic weights. He was apparently the first to assign hydrogen the weight of "1." Newlands noted that properties repeated when the sequence was broken into periods of seven and referred to his system as the "law of octaves."
During the mid-1860s Meyer took the newly established atomic weights of many elements and arranged them into families that bore similarities in properties, including the ability of an atom to combine with other atoms (valency).
In 1869 Mendeleev presented his table of the elements (sixty-three by now) arrayed in periods of seven for the lighter elements and opening up to seventeen for the heavier elements. Furthermore, Mendeleev had the foresight and confidence to break the atomic weight sequence by occasionally forcing elements out of order so as to fall in an appropriate location as determined by their properties. He left gaps in the arrangement at several places and used implied trends to predict characteristics of undiscovered elements needed to complete the table.
Mendeleev's Periodic Table was not well received at first, but was shortly helped by the discovery of the element gallium, which filled such a gap and had nearly exactly the atomic weight, density, and valency predicted. Other affirmations followed. It is for these reasons that Mendeleev is given most of the credit for the invention of the Periodic Table.
In 1892 Scottish chemist William Ramsay discovered two more elements, argon and helium. These unreactive gases did not fit into the Periodic Table. In short order, Ramsay also discovered three more unreactive gases. These gases represented a new family of elements that had to be inserted as an eighth main column in Mendeleev's table.
The Modern Periodic Table
As the twentieth century approached, elements of similar properties were arranged in eight main vertical columns referred to as chemical families. The first such family, or Group I, is collectively termed the "alkali metals," commencing with lithium. The next column, Group II, is designated the "alkaline earths," commencing with beryllium. Groups III through V are commonly referred to as the boron, carbon, and nitrogen families, respectively. The next group, the oxygen family, is technically called the "chalcogens." Group VII, the "halogens , " begins with fluorine. Finally, the elements of Group VIII, starting with helium, are called the "noble gases." Because of their relative unreactivity, they had once also been termed inert gases, a label no longer acceptable.
In the absence of any understanding as to why the periodic arrangement appeared as it did, or whether or not there were yet more surprises, the science of chemistry remained incomplete, although very important and practical. At almost exactly this time, just before the start of the twentieth century, three findings were announced that changed the course of science: x rays were discovered by German physicist Wilhelm Röntgen in 1895, radioactivity by French physicist Antoine-Henri Becquerel in 1896, and the electron by British physicist Joseph John Thomson in 1897.
First Model
What soon emerged was a nuclear model of the atom, first proposed by New Zealand-born physicist Ernest Rutherford. In this view, an element's identity was determined by its atomic number, the amount of positive charge in the very small core nucleus that also contained almost all of the atom's mass. The light electrons were held in orbits by electrostatic attraction to the positive core.
Rutherford's view was extended by Danish physicist Niels Bohr in 1913. Bohr modeled that electrons moved in fixed orbits around the nucleus, much as planets orbit the Sun. Furthermore, not only were the locations of these orbits fixed, but so were the speeds of the electrons in each orbit and the number of electrons that could be accommodated in each orbit, a description called the electron configuration. By explaining the quota of electrons allowed in each fixed orbit, Bohr resorted to a new physical idea called quantization. As a consequence, Bohr was able to reproduce the Periodic Table, adding one electron at a time as one stepped to the next higher element. Bohr argued that orbits of increasing radius could accommodate up to a maximum number of electron numbers that, when reached, corresponded to observed horizontal periods of two, eight, eight, eighteen, eighteen, and thirty-two. Bohr acknowledged the unattractiveness of this approach in that it was merely mimicking an observed pattern rather than addressing the underlying science.
Modern Theory
The mid-1920s witnessed a necessary breakthrough. The revolutionary wave concept of matter was incorporated into a mathematical framework, a new quantum theory, that explained all the properties of a bound electron: its energy, the description of where it could be found, and configuration restraints.
An electron could have only certain energies determined by the value of an integer (a whole number), traditionally symbolized by n with values 1, 2, 3, and so on. Electron energy with n = 1 is the lowest possible, n = 2 being the next lowest, and so on. The region of space where the electron might be found—called an orbital because it replaced Bohr's planetary fixed orbit idea—could be characterized by its size, shape, and orientation (how the shape might be tilted). For each n , there was a determined set of shapes and orientations with letters used to indicate the shapes. For n = 1, only a spherical shape is allowed, symbolized by s ; since a sphere has no orientation, that is the only n = 1 orbital. It is abbreviated as 1s. For n = 2, there are larger orbitals: another s , the 2s , and also dumbbell-shaped orbitals with opposing lobes. These are symbolized as p orbitals and have three possible orientations for the 2p and all other p orbitals. By the time n = 3 is considered, there is a third shape, d, with five orientations. For n = 4 there is a fourth shape, f, with seven orientations in addition to the 4s, 4p and 4d. The sequence of filling follows a relatively simple pattern shown by arrows in Figure 1.
Very early in the development of modern quantum mechanics, German physicist Wolfgang Pauli realized that each of the substates characterized by n, shape, and orientation was permitted to have no more than two electrons, a feature sometimes pictured as if the electron were spinning and where only two spin orientations were allowed: clockwise and counterclockwise.
The predicted sequence of electron filling might be best illustrated by looking at some examples. Hydrogen is 1s 1 , the superscript referring to the number of electrons in the 1s substate. Lithium (three electrons) and sodium (eleven electrons) are 1s 2 2s 1 and 1s 2 2s 2 2p 6 3s 1 , respectively. The latter configuration, for example, corresponds to one pair of 1s- electrons, one pair of
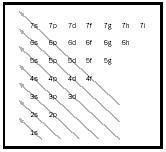
2s- electrons, three pairs of 2p- electrons (six total), and a final 3s -electron. Neon and argon are 1s 2 2s 2 2p 6 and 1s 2 2s 2 2p 6 3s 2 3p 6 , respectively. They complete the horizontal periods of length eight.
The periods in which the d substates are filling are known as the d- block elements or transition metals . These ten elements increase the period length to eighteen elements. Some new Periodic Tables have adopted the convention of numbering the columns one through eighteen as a result.
The f- block, whose existence was recognized by American chemist Glenn Seaborg, has two rows containing nearly one-quarter of all the elements. The first row is known as the rare earth elements or lanthanides . The second f- block row is referred to as the actinides. The most common form of the Periodic Table, the Mendeleev-Seaborg form, has the f- elements at the bottom. Fourteen f- block elements increase the period length to thirty-two.
For nearly three centuries, a new element has been discovered every two and-one-half years, on average. Undoubtedly, more will be found. Although their names and their discoveries will likely involve controversies, their place at the table is already set.
SEE ALSO Alchemy ; Avogadro, Amedeo ; Becquerel, Antoine-Henri ; Bohr, Niels ; Cannizzaro, Stanislao ; Dalton, John ; Lavoisier, Antoine ; Mendeleev, Dimitri ; Meyer, Lothar ; Pauli, Wolfgang ; Ramsay, William ; RÖntgen, Wilhelm ; Rutherford, Ernest ; Seaborg, Glenn Theodore ; Thomson, Joseph John .
Paul J. Karol
Bibliography
Marshall, James L. (2000). "A Living Periodic Table." Journal of Chemical Education 77:979–983.
Mazurs, Edward G. (1974). Graphic Representations of the Periodic System during One Hundred Years, revised 2nd edition. University: University of Alabama Press.
van Spronsen, J. W. (1969). The Periodic System of Chemical Elements: A History of the First Hundred Years. New York: Elsevier.
Internet Resources
Winter, Mark. "WebElements Period Table." The University of Sheffield and WebElements Ltd., U.K. Available from http://www.webelements.com .
Comment about this article, ask questions, or add new information about this topic: