Green Chemistry

Green chemistry is also known as environmentally benign chemistry, or sustainable chemistry. Perhaps the most widely accepted definition of green chemistry is the one offered by chemists Paul Anastas and John Warner, who defined green chemistry as the design of chemical products and processes that reduce or eliminate the use and generation of hazardous substances.
Milestones
The Pollution Prevention Act of 1990 set the stage for green chemistry: Its focus is the prevention of pollution at the source rather than the treatment of pollutants after they are formed. This goal became a formal objective of the Environmental Protection Agency (EPA) in 1991. Anastas coined the term "green chemistry" the same year. Two of the most prominent and early advocates of green chemistry were Kenneth Hancock of the National Science Foundation (NSF) and Joe Breen, who after twenty years of service at the EPA then became the first director of the Green Chemistry Institute (GCI) during the late 1990s.
Anastas and Warner formulated the twelve principles of green chemistry in 1998. These serve as guidelines for chemists seeking to lower the ecological footprint of the chemicals they produce and the processes by which such chemicals are made.
Starting in 1996, outstanding examples of green chemistry have been recognized in the United States each year by the Presidential Green Chemistry Challenge (PGCC) awards. These are the only awards in chemistry that are bestowed at the presidential level.
The EPA and the American Chemical Society (ACS) have played a major role in promoting research and development, as well as education, in green chemistry. In 2000 the GCI became a partner of the ACS. Chemical societies around the globe have recognized the importance of green chemistry and promote it through journals, conferences, educational activities, and the formation of GCI chapters. There are GCI chapter affiliates all over the world.
Importance to Industry: The Triple Bottom Line
During the 1990s many industries began to earnestly adopt green chemistry and other sustainable practices. Forward-looking companies realized that the practice of green chemistry not only leads to environmental benefits, but also economic and social benefits. The combination of these three benefits is known as the "triple bottom line" and provides strong encouragement for businesses to develop sustainable products and processes.
The following real-world examples of green chemistry represent the accomplishments of several winners of the PGCC awards. They illustrate how green chemistry impacts a wide array of fields including pharmaceuticals, pesticides, polymers, and many others.
The Concept of Atom Economy
When chemists are considering a compound, they are concerned with the chemical, biological, and physical properties of this compound, and the
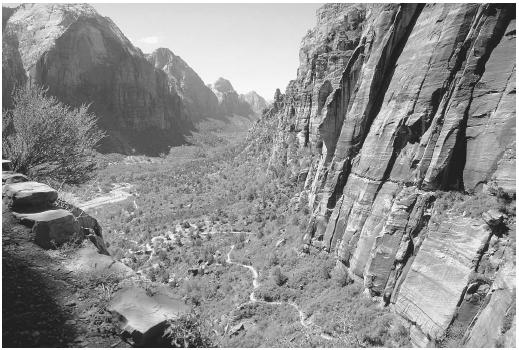
method by which the compound is prepared or its synthesis . In order to focus greater attention on waste by-products that are formed during a synthesis, Barry Trost of Stanford University developed the concept of atom economy. This concept deals with the question: How many of the atoms of the reactants are incorporated into the final desired product and how many are wasted by incorporation into by-products? An example of the application of this concept is discussed in the following synthesis of ibuprofen.
Pharmaceuticals. Ibuprofen is the active ingredient in many analgesic and inflammatory drugs such as Advil, Motrin, and Medipren. Beginning in the 1960s, ibuprofen was produced by a six-step synthesis with an atom economy of only 40 percent. This meant that less than half (40 percent) of the weight of all the atoms of the reactants were incorporated in the ibuprofen, and 60 percent were wasted in the formation of unwanted by-products. The annual production of approximately 30 million pounds of ibuprofen by this method resulted in over 40 million pounds of waste. But during the 1990s, the BHC Company developed a new synthesis of ibuprofen with an atom economy of 77 to 99 percent. This synthesis not only produces much less waste, it is also only a three-step process. A pharmaceutical company can thus produce more ibuprofen in less time and with less energy, which results in increased profits.
Pesticides. Dichlorodiphenyl trichloroethane (DDT) is one of the most well-known insecticides. During World War II it saved thousands of Allied lives by killing disease-carrying insects, but during the 1960s the significant environmental damage caused by DDT was brought to the public's attention by Rachel Carson in Silent Spring (1962). As a result of the controversy generated by this book and other media coverage, the substance's use was banned in the United States in 1973.
During the 1960s and 1970s organophosphates largely replaced organo-chlorine pesticides such as DDT. These pesticides rapidly degrade in the environment, but they are much more toxic to mammals. They are deadly to a wide array of insects and kill not only the target organism but also beneficial insects, such as bees and predatory beetles, and can also be harmful to humans.
One approach to producing less environmentally harmful pesticides is to use compounds that destroy only the target organisms. One manufacturer, Rohm & Haas, has developed insecticides that mimic a hormone used only by molting insects. Insects that do not molt are not affected, leaving many beneficial insects unharmed.
A more recent strategy for protecting plants from pests and disease involves the use of genetically altered plants. This method is controversial. Concerns include cross-pollination with unaltered plants and the entry of altered plants into the food supply.
THE TWELVE PRINCIPLES OF GREEN CHEMISTRY
1. It is better to prevent waste than to treat or clean up waste after it is formed.
2. Synthetic methods should be designed to maximize the incorporation of all materials used in the process in the final product.
3. Wherever practical, synthetic methodologies should be designed to use and generate substances that possess little or no toxicity to human health and the environment.
4. Chemical products should be designed to preserve efficacy of function while reducing toxicity.
5. The use of auxiliary substances (e.g., solvents, separation agents, etc.) should be made unnecessary whenever possible and innocuous when used.
6. Energy requirements should be recognized for their environmental and economic impacts and should be minimized. Synthetic methods should be conducted at ambient temperature and pressure.
7. A raw material feedstock should be renewable rather than depleting whenever technically and ecomonically practical.
8. Unnecessary derivatization (blocking group, protection/deprotection, temporary modification of physical/chemical processes) should be avoided whenever possible.
9. Catalytic reagents (as selective as possible) are superior to stoichiometric reagents.
10. Chemical products should be designed so that at the end of their function they do not persist in the environment and break down into innocuous degradation products.
11. Analytical methodologies need to be further developed to allow for real-time in-process monitoring and control prior to the formation of hazardous substances.
12. Substances and the form of a substance used in a chemical process should be chosen so as to minimize the potential for chemical accidents, including releases, explosions, and fires.
Source: Anastas, Paul T., and Warner, John C.(1998). Green Chemistry Theory and Practice. New York: Oxford University Press.
Another approach to protecting plants from pests and diseases is to activate their natural defense mechanism against pests or diseases. EDEN Bioscience Corporation has developed what is known as harpin technology. Harpin is a naturally occurring protein that is isolated from genetically altered bacteria. When applied to the leaves and stems of plants, this protein elicits their natural defense systems. The EPA has classified harpin as Category IV, which is reserved for materials with the lowest hazard potential. As an added benefit, harpin also stimulates plant growth.
Polymers. Synthetic polymers or plastics are everywhere. They are used in cars, computers, planes, houses, eyeglasses, paints, bags, appliances, medical devices, carpets, tools, clothing, boats, batteries, and pipes. More than 60 million pounds of polymers are produced in the United States each year. The feedstocks that are used to produce these polymers are virtually all made from petroleum, a nonrenewable resource. Approximately 2.7 percent of all crude oil is used to generate chemical feedstocks.
In order to decrease human consumption of petroleum, chemists have investigated methods for producing polymers from renewable resources such as biomass . NatureWorks polylactic acid (PLA) is a polymer of naturally occurring lactic acid (LA), and LA can be produced from the fermentation of corn. The goal is to eventually manufacture this polymer from waste biomass. Another advantage of PLA is that, unlike most synthetic polymers which litter the landscape and pack landfills, it is biodegradable. PLA can also be easily recycled by conversion back into LA. It can replace many petroleum-based polymers in products such as carpets, bags, cups, and textile fibers.
Computer Chips. The manufacture of computer chips requires excessive amounts of chemicals, water, and energy. Estimates indicate that the weight of chemicals and fossil fuels required to make a computer chip is 630 times the weight of the chip, as compared to the 2:1 ratio for the manufacture of an automobile. Scientists at the Los Alamos National Laboratory have developed a process that uses supercritical carbon dioxide in one of the steps in chip preparation, and it significantly reduces the quantities of chemicals, energy, and water needed to produce chips.
Dry Cleaning. Condensed phase carbon dioxide is also used as a solvent for the dry cleaning of clothes. Although carbon dioxide alone is not a good solvent for oils, waxes, and greases, the use of carbon dioxide in combination with a surfactant allows for the replacement of perchloroethylene (which is the solvent used most often to dry clean clothes, although it poses hazards to the environment and is a suspected human carcinogen).
Other Examples. Some other examples of green chemistry include the following:
- taking chromium and arsenic, which are toxic, out of pressure-treated wood
- using new less toxic chemicals for bleaching paper
- substituting yttrium for lead in auto paint
- using enzymes instead of a strong base for the treatment of cotton fibers
Green chemistry reduces toxicity, minimizes waste, saves energy, and cuts down on the depletion of natural resources. It allows for advances in chemistry to occur in a much more environmentally benign way. In the future, when green chemistry is practiced by all chemists and all chemicalrelated companies, the term "green chemistry" will ideally disappear as all chemistry becomes green.
SEE ALSO Ibuprofen ; Industrial Chemistry, Organic ; Solution Chemistry .
Michael C. Cann
Bibliography
Anastas, Paul T., and Warner, John C. (1998). Green Chemistry Theory and Practice. New York: Oxford University Press.
Cann, Michael C., and Connelly, Marc E. (2000). Real-World Cases in Green Chemistry. Washington, DC: American Chemical Society.
Kirchhoff, Mary, and Ryan, Mary Ann, eds. (2002). Greener Approaches to Undergraduate Chemistry Experiments. Washington, DC: American Chemical Society.
Ryan, Mary Ann, and Tinnesand, Michael, eds. (2002). Introduction to Green Chemistry. Washington, DC: American Chemical Society.
Internet Resources
Green Chemistry Institute Web site. Available from http://chemistry.org/greenchemistryinstitute\ .
University of Scranton. "Green Chemistry." Available from http://academic.scranton.edu/faculty/CANNM1/greenchemistry.html .
U.S. EPA. "Green Chemistry." Available from http://www.epa.gov/greenchemistry/index.html .
Comment about this article, ask questions, or add new information about this topic: