Superconductors
Superconductivity, which is defined as the absence of resistance in a conducting material to a continuously flowing electric current, is a special property that a sizable number of substances attain suddenly at very low temperatures. The substances (called superconductors) include elements, alloys , compounds, and nonstoichiometric ceramic materials. Superconductors also exhibit perfect diamagnetism; that is, magnetic fields cannot penetrate them (the Meissner effect), and small powerful magnets actually float (levitate) above flat superconductor surfaces. A superconductor's critical transition temperature, T C , is the temperature above which no super-conductivity can be obtained. For elements, alloys, and simple compounds, very low critical transition temperatures ( T C 23 K) mean that the cooling effects of liquid helium ( B.P. = 4 K) are needed to bring about and to maintain their superconductivity. The discovery in 1986 that nonstoichiometric ceramics containing copper and oxygen can have much higher T C values has provided a new impetus for developing superconducting materials.
High Temperature Superconductors
In April 1986, K. Alex Müller and J. Georg Bednorz (with IBM in Switzerland) reported the superconductivity of a nonstoichiometric ceramic oxide of lanthanum, barium, and copper, La 2− x Ba x CuO 4− y , with the then record high T C of 35 K. Further experiments conducted by Müller, Bednorz, and others showed that slight modifications made to La 2− x Ba x − CuO 4− y ( x 0.2 and y is even smaller) could yield materials having T C s of 50 K. By early 1987, Paul C. W. Chu (at the University of Houston), Maw-Kuen Wu (at the University of Alabama), and their coworkers synthesized another ceramic oxide material, YBa 2 Cu 3 O 7− y , and observed that super-conductivity in the material was attainable by cooling it with liquid nitrogen ( B.P. = 77 K). This "high temperature superconductor" made possible
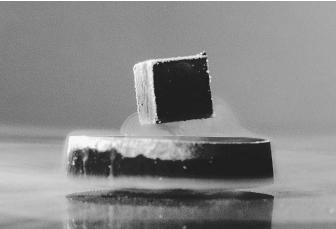
superconductor applications that were impractical with the low temperature superconductors. (See Figure 1.)
Other nonstoichiometric ceramic oxides that contain copper in nonintegral oxidation states have been synthesized and evaluated. Several of these materials have even higher T C 's than that of YBa 2 Cu 3 O 7− y . The BSCCO series Bi 2 Sr 2 Ca n − 1Cu n O 2 n +4+ y (for n = 1 to 4) reaches a T C maximum of 110 K for Bi 2 Sr 2 Ca 2 Cu 3 O 10+ y ; a similar Tl 2 Ba 2 Ca n −1Cu n O 2 n +4+ y series reaches a maximum of 122 K for Tl 2 Ba 2 Ca 2 Cu 3 O 10+ y ; HgBa 2 Ca 2 Cu 3 O 8+ y has a T C of 135 K at ambient pressure; and Hg 0.8 Tl 0.2 Ba 2 Ca 2 Cu 3 O 8.33 has a T C of 138 K. Also, the T C of HgBa 2 Ca 2 Cu 3 O 8+ y has been reported to increase to 153 K at a pressure of 150,000 atmospheres and to 160 K at 280,000 atmospheres. Even higher T C values have been claimed for portions of multiparticle ceramics, but no macroscopic material has shown unambiguous superconductivity at these higher temperatures (above 160 K).
Other new classes of superconductors that are being investigated include intermediate temperature range superconductors, such as magnesium diboride ( T C = 39 K), alkali-doped C 60 (M 3 C 60 has a T C of 33 K), and hole-doped C 60 ( T C = 52 K). The latter result led Jan Hendrik Schon, Christian Kloc, and Bertram Batlogg (of Bell Labs) to the newer haloform-intercalated, high temperature C 60 superconductors C 60 · 2CHCl 3 and C 60 · 2CHBr 3 , with T C values of 80 K and 117 K, respectively.
The theoretical interpretation of the high temperature superconductors is still under development. The copper oxide ceramic superconductors obtain their paired conducting electrons from copper in mixed oxidation states of I and II or II and III, depending on the particular system. The paired conducting electrons are called Cooper pairs, after Leon N. Cooper. Cooper's name also gives us the C of BCS; the BCS theory is an interpretation of superconductivity for low temperature superconductors (having T C 's of less than 40 K).
MEISSNER EFFECT
The Meissner effect is the repulsion of a magnetic field from the interior of a super-conductor below its critical temperature. Whereas a weak magnetic field is totally excluded from the interior of a superconductor, a very strong magnetic field will penetrate the material and concurrently lower the critical transition temperature of the superconductor. W. Meissner and R. Ochsenfeld discovered the Meissner effect in 1933.
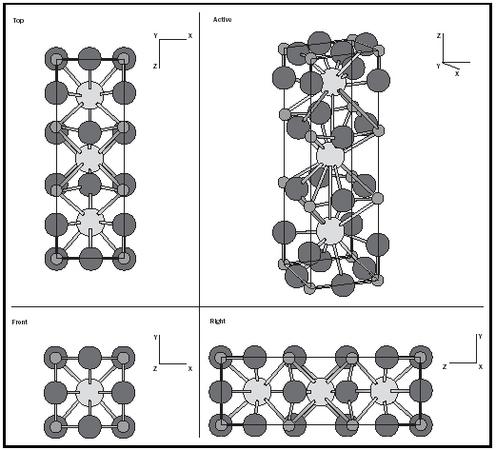
Applications
Applications for superconducting materials include strong superconducting magnets without iron cores, which in turn have a variety of uses. These superconducting magnets are used in particle accelerators, nuclear magnetic resonance and magnetic circular dichroism instruments, magnetic resonance imaging devices in medicine, levitating trains, magnetic refrigerators, magnetic energy storage, and SQUIDS (superconducting quantum interference devices) for very sensitive magnetic field measurements (including biomedical magnetoencephalography). Most magnetic applications were developed with and still use the older low temperature superconductors, such as niobium-titanium alloy superconducting wire, which must be cooled with liquid helium. For example, the Fermilab Tevatron (1983) uses 1,000 liquid helium–cooled superconducting magnets in its four-mile (circumference) proton-antiproton collider. The sudden quenching of superconducting magnets by vibrations, external fields, or accidental warming can be a serious problem, and the associated heat can cause extensive helium loss.
Cooling with liquid nitrogen rather than liquid helium is much more economical. The difficulty in molding the high temperature superconductors into strong and flexible forms (e.g., filaments or wires) and the greater T C lowering that accompanies greater magnetic field strength have limited their use up to the present time. Several firms have developed methods to improve the transfer of charge among superconducting particles; and it appears that the best superconductors may be impure ones that allow (the more disordered) ceramic glass formation rather than ceramic crystallite formation. BSCCO superconducting transmission lines are being manufactured (by American Superconductor, Pirelli, and Intermagnetics General) but are currently competitive only when space or weight limitations are important. Superconducting filters for cellular communications, in which the lack of resistance provides filters that have minimal signal loss and are more discriminating in frequency tuning, are being marketed (by Superconductor Technologies, Illinois Superconductor [ISCO], and Conductus), and superconducting motors (American Superconductor) and generators (General Electric) are under development.
BCS THEORY
BCS theory, developed by John Bardeen, Leon Cooper, and Robert Schrieffer, provides complicated mathematical equations that satisfactorily explain the superconductivity of the classical low temperature superconductors with critical transition temperatures below 40 K. The theory includes Cooper pairs of electrons but does not explain the high critical transition temperatures of the newer ceramic superconductors.
SEE ALSO Ceramics ; Cryogenics ; Magnetism .
Ronald D. Archer
Bibliography
Cava, Robert J. (1990). "Superconductors Beyond 1–2–3." Scientific American 263(2): 42–49.
Chu, Paul C. W. (1995). "High-Temperature Superconductors." Scientific American 273(3): 162–165.
Dinnebier, Robert E.; Gunnarsson, Olle; Brumm, Holger; et al. (2002). "Structure of Haloform Intercalated C 60 and Its Influence on Superconductive Properties." Science 296(1): 109–113.
Müller, K. Alex, and Bednorz, J. Georg (1987). "The Discovery of a Class of High-Temperature Superconductors." Science 237: 1,133–1,139.
Schechter, Bruce (2000). "No Resistance: High-Temperature Superconductors Start Finding Real-World Uses." Scientific American 283(2): 32–33.
Wolsky, Alan M.; Giese, Robert F.; and Daniels, Edward J. (1989). "The New Superconductors: Prospects for Applications." Scientific American 260(2): 60–69.
Internet Resources
Eck, Joe. "Superconductor Information for the Beginner." Available from http://superconductors.org .
Naval Research Laboratory. Center for Computational Materials Science. More information. Crystal structure of YBa 2 Cu 3 O 7-y . Available from http://cst-www.nrl.navy.mil/lattice/struk.picts/ .
"Perovskite Structures." Naval Research Laboratory. Center for Computational Materials Science. Available from http://cst-www.nrl.navy.mil/lattice/struk/ .
Comment about this article, ask questions, or add new information about this topic: