Solution Chemistry
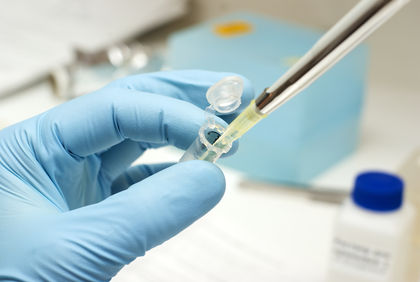
The majority of chemical processes are reactions that occur in solution. Important industrial processes often utilize solution chemistry. "Life" is the sum of a series of complex processes occurring in solution. Air, tap water, tincture of iodine, beverages, and household ammonia are common examples of solutions.
A solution is a homogenous mixture of substances with variable composition. The substance present in the major proportion is called the solvent, whereas the substance present in the minor proportion is called the solute. It is possible to have solutions composed of several solutes. The process of a solute dissolving in a solute is called dissolution.
Many common mixtures (like concrete) are heterogeneous —the components and properties of such mixtures are not distributed uniformly throughout their structures. Conversely, solutions are said to be homogeneous because they have uniform composition and properties. Solutions are intimate and random homogeneous mixtures of atomic-size chemical species, ions, or molecules.
In addition to their observed homogeneity, true solutions also have certain other characteristics. For example, components of a solution never separate spontaneously, even when a significant density difference exists between the components. Solutions also pass through the finest filters unchanged.
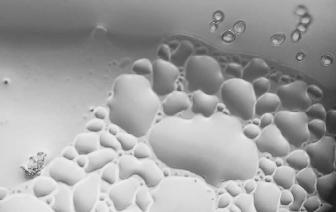
The components of a solution distribute themselves in a completely random manner, given sufficient time. For example, a lump of sugar dropped into a glass of water dissolves, and eventually molecules of sugar can be found randomly distributed throughout the water, even though no mechanical stirring has been employed. This phenomenon, called diffusion, is similar to the process of diffusion that occurs with gases. The molecules of sugar (as well as those of water) must be in constant motion in the solution. In the case of liquid solutions, the sugar molecules do not move very far before they encounter other molecules; diffusion in a liquid is therefore less rapid than diffusion in a gas.
Kinds of Solutions
Many commonly encountered solutions are those involving a solid that has been dissolved in a liquid, but there are as many types of solutions as there are different combinations of solids, liquids, and gases. Solutions in which the solvent is a liquid and the solute is a gas, liquid, or solid are very common. The atmosphere is a good example of a solution in which a gaseous solvent (nitrogen) dissolves other gases (such as oxygen, carbon dioxide, water vapor, and neon). Solutions of solids in solids are another example, and these are encountered most often among the various metal alloys.
Of all the liquid solvents used in the laboratory, in industry, and in the home, water is the most commonly employed and is the best of the inorganic solvents. The alcohols and numerous other types of compounds are classified as organic solvents; many of these are used in dry cleaning chemicals, nail polish removers, paint thinners, and many other similar purposes.
Concentration
The concentration of a solution is defined as the amount of solute present in a given quantity of solvent. Very often scientists speak of concentrated solutions, dilute solutions, or very dilute solutions, but these designations give only a rough relative qualitative idea of concentration. For example, a "concentrated solution" contains a considerable quantity of solute as compared with a "dilute solution." Although such designations are only qualitatively useful, they are nevertheless widely used.
The most common way to express concentration is on the basis of the weight of solute per unit weight of solvent. For example, a salt solution may be prepared by dissolving 1.64 grams of sodium chloride in 100 grams of water. The concentration of this solution could also be expressed as 0.0164 grams of NaCl per 1 gram of water, or as 16.4 grams of NaCl per 1,000 grams of water. Thus, a statement of the concentration of a solution does not imply anything concerning the amount of solute or the amount of solvent present, but rather gives the ratio of solute to solvent in terms of some convenient (and arbitrary) units. Because the weight of a sample of a liquid is usually more difficult to determine experimentally than its volume, a practical unit of concentration is the weight of solute in a given volume of the solution; for example, a sugar solution may contain 50 grams of sugar per 100 milliliters of solvent.
Solubility
Solubility is a measure of the maximum amount of solute that can be dissolved in a given amount of solvent to form a stable solution. The composition of many solutions cannot be varied continuously because there are certain fixed limits imposed by the nature of the substances involved. Solid salt and sugar can be mixed in any desired proportions, but unlimited quantities of sugar (or salt) cannot be dissolved in a given amount of water; however, up to the solubility limit, solutions can be produced in any desired proportion.
When the solvent contains a maximum quantity of solute, the resulting solution is said to be saturated. The saturation point varies according to the solute. For example, 100 grams of pure water at 25°C (77°F) can dissolve no more than 35.92 grams of NaCl to form a stable saturated solution, but this same amount of water at 25°C dissolves only 0.0013 grams of calcium carbonate. The solubility in these examples is expressed in grams of solute per 100 grams of water, but any suitable units could be used. Water can dissolve any amount of a solute less than that required for a saturated solution. Tables of the solubilities of many substances can be found in various chemistry texts.
In some cases there is no upper limit to the amount of a solute that a given quantity of solvent can dissolve, and these substances are said to be miscible in all proportions. Completely miscible substances give homogeneous mixtures (solutions); for example, a mixture of any two gaseous substances is homogeneous. Often, liquids such as alcohol and water can be mixed in all proportions to give homogeneous mixtures.
When a saturated solution has been achieved, a dynamic equilibrium exists between the solute in solution and any undissolved solute. Molecules of the solute (or atoms or ions, depending upon the nature of the solute) are continuously going into solution, but since the solution is already saturated, an equal number of molecules of the solute leave the solution and redeposit on the excess solid solute. A state of equilibrium exists when these two processes occur at the same rate, the net result being a constant amount of solute in solution. A saturated solution can therefore be defined more precisely as a solution that is in equilibrium with an excess of the solute at a given temperature.
In some instances it is possible to prepare a true solution that contains an excess of the equilibrium amount of solute; this condition is called supersaturation. Supersaturated solutions are unstable. If left undisturbed, they may remain in this state for an indefinite period of time. However, the excess solute can be brought out of solution by a slight agitation or by the addition of any solid particle (dust, a small crystal of solute, etc.) that can act as a center for crystal growth, returning the solution to its normal saturated state.
Conditions That Affect Solubility
In general, three major factors—pressure, temperature, and the nature of the solute and solvent—influence the solubility of a solute in a solvent. Not all these factors are equally important in a specific instance.
Pressure. Changes in pressure have little effect on the solubility of solid or liquid solutes in a liquid solvent, but pressure has a much greater influence on the solubility of a gaseous solute. A commonly observed phenomenon that supports this is the effervescence that occurs when the cap of a bottle of ordinary soda water is removed. Soda water contains carbon dioxide gas dissolved in water under pressure; when the cap is removed, the pressure of the gas on the liquid is decreased to atmospheric pressure. Since carbon dioxide gas leaves the solution at this lower pressure, it follows that the solubility of carbon dioxide in water is dependent upon the pressure of the carbon dioxide above the liquid. The results of this simple observation are summarized in Henry's Law, which states that at any specified temperature, the extent to which a gas dissolves in a liquid is directly dependent upon the pressure of the gas.
Temperature. In general, a change in temperature affects the solubility of gaseous solutes differently than it does the solubility of solid solutes, because the solubility of a gas in a liquid solvent decreases with increasing temperature. With relatively few exceptions, the solubility of solids in liquids increases with an increase in temperature. In some instances, the increase in solubility is very large; for example, the solubility of potassium nitrate in water at 25°C is about 31 grams of KNO 3 per 100 grams of water and about 83 grams of KNO 3 per 100 grams of water at 50°C (122°F). On the other hand, the solubility of some solutes, such as ordinary table salt, shows very little dependence on temperature. Often this difference in solubility can be used as an advantage in the preparation, isolation, or purification of substances by the process of crystallization. In general, it is not possible to arrive at any reliable generalization concerning the influence of temperature upon the solubility of liquids in liquids. In some cases the solubility increases with an increase in temperature, in some cases it decreases, and in others there is very little effect.
The nature of solute and solvent. Crystalline substances consist of a regular arrangement of atoms, molecules, or ions; in the latter case, the forces that hold the crystal together are electrostatic in nature. For an ionic crystal to dissolve in water, the water molecules must be able to shield the charges of the positive and negative ions from each other. The attractive forces between the ions in solution are less than those in the solid state because of the solvent molecules; hence, the ions behave more or less independently in solution. In general, the relative solubilities of ionic substances are a measure of the magnitude of the electrostatic forces that hold the crystals together.
Properties of Solutions
Pure liquids have a set of characteristic physical properties (melting point, vapor pressure at a given temperature, etc.). Solutions in a solvent exhibit these same properties, but the values differ from those of the pure solvent because of the presence of the solute. Moreover, the change observed in these properties in going from the pure solvent to a solution is dependent only upon the number of solute molecules; these properties are called colligative properties. The properties of a solvent that show a predictable change upon the addition of a solute are melting point, boiling point, vapor pressure, and osmotic pressure.
Melting and boiling points. Solutions exhibit higher boiling points and lower melting points than the parent solvent. The increase in boiling point and decrease in melting point is dependent upon the number of solute particles in the solution. The greater the number of solute particles (i.e., the concentration), the greater the boiling point elevation and melting point depression. A common application of this effect in some parts of the world is in the use of antifreeze solutions in the cooling systems of automobiles in cold climates. "Antifreeze" compounds are usually organic liquids that are miscible with water so that large freezing point effects can be attained.
Vapor pressure All liquids exhibit a vapor pressure, the magnitude of which depends on the temperature of the liquid. For example, water boils at 100°C, which means that at 100°C the vapor pressure of water is equal to the atmospheric pressure allowing bubbles of gaseous water (steam) to escape from the liquid state. However, the vapor pressure of a solution (at any temperature) is less than that of the solvent. Thus, boiling water ceases to boil upon the addition of salt because the salt solution has a lower vapor pressure than pure water. The salt solution will eventually boil when the temperature of the solution increases bringing about an increase in vapor pressure sufficient to again form bubbles. Note in this example that the boiling point of water increases with the addition of salt; thus, the boiling point elevation and the vapor pressure depression are related.
Osmosis. This property of solutions is perhaps the least familiar of the colligative properties, but in a sense it is more important than those already mentioned. In 1748 French clergyman and physicist Jean-Antoine Nollet observed that certain animal membranes are selectively permeable to different molecules. Since then, many examples of semipermeable membranes have been discovered, including animal bladder or gut tissues, eggshell lining, and certain vegetable tissues. A semipermeable membrane may be defined as a material that allows molecules of one kind to pass through it but prevents the passage of other kinds of molecules or allows the passage of different kinds of molecules at different rates. Membranes often permit the passage of solvent molecules and prevent the passage of solute molecules. The phenomenon of osmosis is of far-reaching importance in biology, medicine, and related areas. Many animal and vegetable membranes are semi-permeable, and the process of osmosis plays an important role in the transfer of molecules through cell walls in biological processes. Osmosis is responsible in part for the germination of seeds and for the rising of sap into the branches and leaves of trees. The preservative action of sugar solutions (e.g., preserves, jellies) is believed to depend upon osmotic processes; bacteria are literally dehydrated.
SEE ALSO Water .
J. J. Lagowski
Bibliography
Ben-Naim, Arieh (1974). Water and Aqueous Solutions: Introduction to a Molecular Theory. New York: Plenum Press.
Lide, David R., ed. (2003). Handbook of Chemistry and Physics, 84th edition. Boca Raton, FL: CRC Press.
Whitten, K. W.; Davis, R. E.; Peck, M. L.; and Stanley, G. G. (2004). General Chemistry. Pacific Grove, CA: Brooks/Cole.
Comment about this article, ask questions, or add new information about this topic: