Walther Hermann Nernst
GERMAN CHEMIST
1864–1941
Walther Hermann Nernst, born in Briesen, Prussia (now Wabrzezno, Poland), was a pioneer in the field of chemical thermodynamics in a wide range of areas. His most outstanding contributions were his laws for electrochemical cells and his heat theorem, also known as the third law of thermodynamics, for which he was awarded the Nobel Prize in chemistry in 1920.
Nernst first studied physics before he became an assistant in 1887 to German physical chemist Friedrich Wilhelm Ostwald at the University of Leipzig, then the only institute for physical chemistry in Germany. In 1891
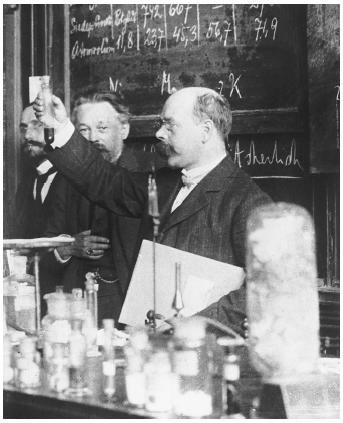
he was appointed associate professor at the university in Göttingen and, three years later, convinced officials there to create an institute for physical chemistry modeled on the Leipzig center. He served as its director until his move in 1905 to Berlin, where he once again established an institute renowned worldwide.
During his Leipzig period, Nernst performed a series of electrochemical studies from which, at the age of twenty-five, he arrived at his well-known equations. These equations described the concentration dependence of the potential difference of galvanic cells, such as batteries, and were of both great theoretical and practical importance. Nernst started with the investigation of the diffusion of electrolytes in one solution. Then he turned to the diffusion at the boundary between two solutions with different electrolyte concentrations; he determined that the osmotic pressure difference would result in an electric potential difference or electromotive force (emf). Next he divided both solutions into two concentration half-cells, connected to each other by a liquid junction, and measured the emf via electrodes dipped into both solutions. The data supported his first equation where the emf was proportional to the logarithm of the concentration ratio. Finally, he investigated galvanic cells where a redox reaction (e.g., Zn + 2Hg + → Zn 2+ + 2Hg) was divided such that oxidation (Zn → Zn 2 + 2 e − ) and reduction (2Hg + + 2 e − → 2Hg) occurred at the electrodes in two half-cells. By combining this with Helmholtz's law, which related thermodynamics to the emf of electrochemical cells, and van't Hoff's equation, which related chemical equilibria to thermodynamics, Nernst derived his second equation for galvanic cells. Supported by many measurements, the equation described the emf of galvanic cells as a function of the concentration of all substances involved in the reaction.
Nernst's formulation of the third law of thermodynamics was originally an ingenious solution to a crucial practical problem in chemical thermodynamics, namely, the calculation of chemical equilibria and the course of chemical reactions from thermal data alone, such as reaction heats and heat capacities. Based on the first two laws of thermodynamics and van't Hoff's equation, chemical equilibria depended on the free reaction enthalpy ΔG , which was a function of both the reaction enthalpy ΔH and the reaction entropy ΔS according to the Gibbs-Helmholtz equation:
The problem was that, although enthalpy values could be calculated from thermal measurements, entropy values required data at the absolute zero of temperature, which was practically inaccessible. Guided by theoretical reasoning and then supported by a huge measurement program at very low temperatures, Nernst in 1906 suggested his heat theorem. According to a later formulation, it stated that all entropy changes approach zero at the absolute zero.
The theorem not only allowed the calculation of chemical equilibria, it was also soon recognized as an independent third law of general thermodynamics with many important consequences. One such consequence was that it is impossible to reach the absolute zero. Another consequence was that one could define a reference point for entropy functions, such that the entropies of all elements and all perfect crystalline compounds were taken as zero at the absolute zero.
Nernst made numerous other important contributions to physical chemistry. For example, his distribution law described the concentration distribution of a solute in two immiscible liquids and allowed the calculation of extraction processes. He also formulated several significant theories, such as those on the electrostriction of ions, the diffusion layer at electrodes, and the solubility product. In addition, he established new methods to measure dielectric constants and to synthesize ammonia, on which the German chemist Fritz Haber later successfully followed up.
SEE ALSO Electrochemistry ; Haber, Fritz ; Ostwald, Friedrich Wilhelm ; Physical Chemistry .
Joachim Schummer
Bibliography
Barkan, Diana (1999). Walther Nernst and the Transition to Modern Physical Science. Cambridge, U.K.: Cambridge University Press.
Hiebert, Erwin N. (1978). "Nernst, Hermann Walther." In Dictionary of Scientific Biography, Vol. XV, Supplement I, ed. Charles C. Gillispie. New York: Scribners.
Mendelssohn, Kurt (1973). The World of Walther Nernst: The Rise and Fall of German Science. London: Macmillan.
Comment about this article, ask questions, or add new information about this topic: