Energy
In the discussion of energy, the fundamental concept is that of work, which is motion against an opposing force. Energy is the capacity to do work. An object traveling at high speed and impacting on another object can do more work—can drive the object farther against an opposing force—than the same object moving slowly. This contribution to energy, the energy ascribed to motion, is called kinetic energy. The kinetic energy of an object of mass m traveling at a speed υ is ½ mυ 2 . An object may also have energy by virtue of its position. An object high above the surface of Earth has more energy (can do more work) than one at its surface. This contribution to the total energy, the energy due to position, is called potential energy. The relation between the object's position and potential energy depends on the nature of the force field it experiences. The potential energy of a body of mass m at a height h above the surface of Earth is mgh, where g is the acceleration of free fall at the location. More important for chemistry is the potential energy of one charge near another charge. The Coulomb potential energy of a charge q 1 at a distance r from a charge q 2 is given by q 1 q 2 /4πϵ 0 r, where ϵ 0 is a fundamental constant called the vacuum permittivity. Energy is also stored in the electromagnetic field in the form of photons. The energy of a photon of radiation of frequency υ is hv, where h is Planck's constant.
Energy is conserved. That is, the sum of the kinetic and potential energies of a single body remains constant provided it is free of external influences (forces). Thus, a falling weight accelerates: The fall implies a reduction of potential energy and the acceleration implies an increase in kinetic energy; the sum, though, is constant. A generalization (which can be interpreted as an implication) of the conservation of energy is the first law of thermodynamics, which focuses on a property of a many-body system called the internal energy. The internal energy can be interpreted as the sum of all the kinetic and potential energies of all the particles comprising the system. The first law of thermodynamics states that the internal energy of an isolated system is constant. The first law is closely related to the conservation of energy, but it acknowledges the possibility of the transfer of energy as heat, which is outside the reach of mechanics itself.
The special theory of relativity states that the mass of a body is a measure of its energy: E = mc 2 , where c is the speed of light. That is, energy and mass are equivalent and interconvertible. Changes in mass are measurable only when changes in energy are considerable, which in practice commonly means for nuclear processes.
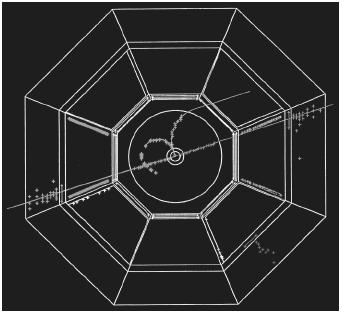
In chemistry we are often concerned with the transfer of energy from one location (e.g., a reaction vessel) to another (the surroundings of that vessel). One mode of transfer is by doing work. For example, work is performed when gases evolved in a reaction push back a movable wall (e.g., a piston) against an opposing force, such as that due to the external atmosphere or a weight to which the piston is attached. Another mode of transfer is as heat. Heat is the transfer of energy that occurs as a result of a temperature difference between a system and its surroundings when the two are separated by a diathermic wall (a wall that allows the passage of energy as heat). A metal wall is diathermic, a thermally insulated wall is not diathermic. Finally, energy may leave a system as electromagnetic radiation, for example as in chemiluminescence—the emission of radiation from matter in energetically excited molecular states produced in the course of a chemical reaction, and as a result of spectroscopic transitions. We shall concentrate on the first two modes of transfer, work and heat.
At a molecular level, work is the transfer of energy that makes use of or drives the orderly motion of molecules in the surroundings. The uniform motion of the atoms in a piston driven back by expanding gas is an example of orderly molecular motion. In contrast, heat is the transfer of energy that makes use of or causes disorderly motion in the surroundings. When we say that a chemical reaction gives out heat, we mean that energy is leaving the reaction vessel and stimulating thermal motion (random molecular motion) in the surroundings.
The energy of a chemical system is stored in the potential and kinetic energies of the electrons and atomic nuclei. This stored energy is sometimes referred to as chemical energy; however, this is only a shorthand way of referring to the kinetic and potential energies of all the particles in an element or compound.
The internal energy of a system changes when a chemical reaction occurs because the electrons and nuclei settle into different arrangements, as in the change of partnerships of H and O atoms in the reaction 2 H 2 (g) + O 2 (g) → 2 H 2 O(g). The energy released in a chemical reaction can be transferred to the surroundings (and put to use) in a variety of ways regardless of the manner in which the energy accumulated in the first place. Thus, energy may escape as heat and be used to raise the temperature of the surroundings, including raising the temperature of water that is then employed in a turbine to do work. The energy may also escape as work. We have already discussed expansion work, using the example of a piston being driven. The work may be accomplished electrically, as when electrons are driven through an external circuit and used to drive an electric motor.
Atomic nuclei are also centers of energy storage as a result of their internal structures. This energy is released when the nucleons (protons and electrons) undergo rearrangement and thereby change the strength of their interactions. The changes in energy are so great that they give rise to measurable changes of mass. For all chemical processes, the changes in mass accompanying acquisition or loss of energy are totally negligible.
SEE ALSO Chemiluminescence ; Chemistry and Energy ; Electrochemistry ; Heat ; Physical Chemistry ; Spectroscopy ; Temperature ; Thermodynamics .
Peter Atkins
Bibliography
Atkins, Peter, and de Paula, Julio (2002). Atkins' Physical Chemistry, 7th edition. New York: Oxford University Press.
Smith, Crosbie (1998). The Science of Energy: A Cultural History of Energy Physics in Victorian Britain. Chicago: University of Chicago Press.
Tipler, Paul Allen (1999). Physics for Scientists and Engineers, 4th edition. New York: W.H. Freeman and Worth Publishers.
Comment about this article, ask questions, or add new information about this topic: